You’re surrounded by them, even if you don’t realize it. They’re humming away inside your phone, your laptop, your car, the server delivering this webpage, and even in large-scale solar farms. We’re talking about DC-DC converters, the unsung heroes of modern electronics.
If you’ve ever been curious about how devices manage power so effectively, how a single battery can power components needing different voltages, or simply want to understand a fundamental building block of power electronics, you’ve come to the right place. This post will dive deep into the world of DC-DC converters: what they are, why they’re indispensable, how they work (without getting too bogged down in heavy math), the different types you might encounter, and where they make their magic happen.
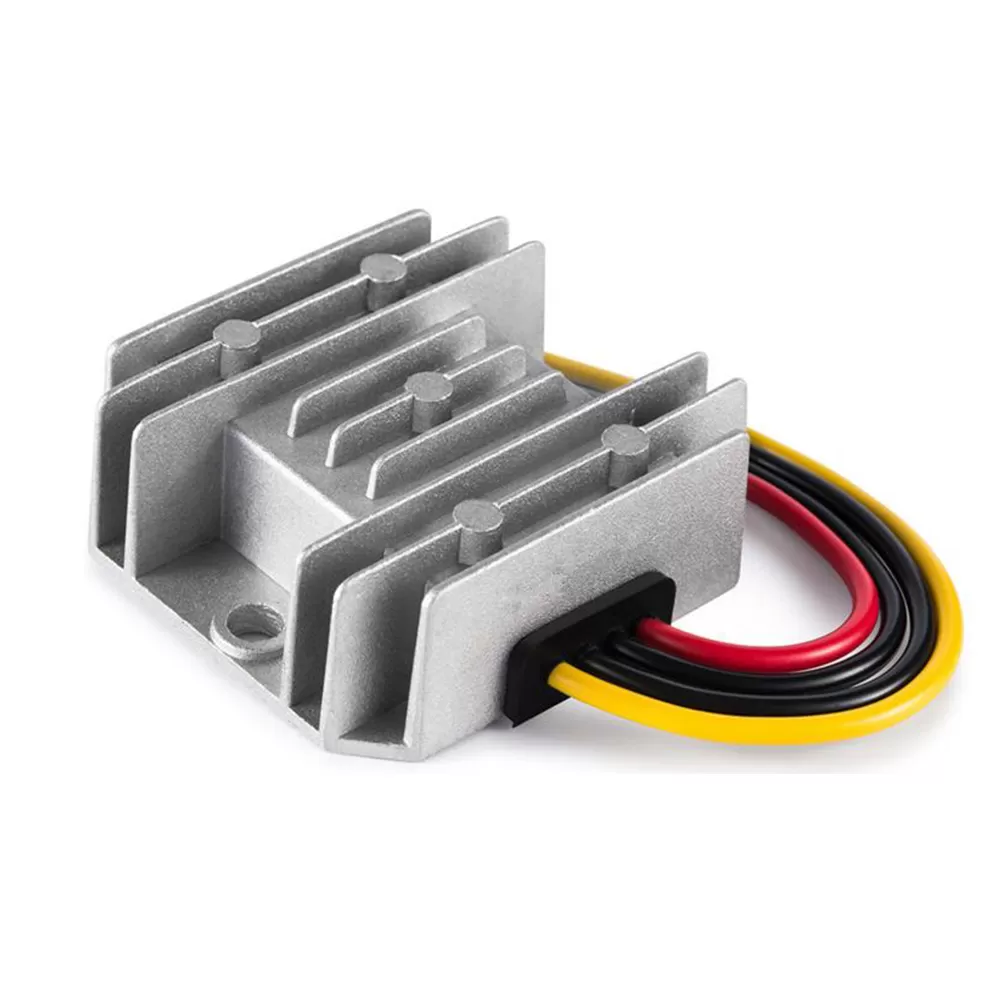
Why Do We Even Need to Convert DC Voltage?
First, let’s tackle the fundamental question: why convert DC (Direct Current) to… well, different DC?
Varying Voltage Requirements: Electronic circuits are complex ecosystems. A single device, like a smartphone, might have a main battery voltage (e.g., 3.7V), but the processor might need 1.1V, the display backlight might need 15V, and other sensors or peripherals could require 3.3V or 5V. A DC-DC converter efficiently generates these multiple voltage “rails” from a single source.
Battery Voltage Fluctuation: Batteries don’t provide a constant voltage. A fully charged lithium-ion battery might output 4.2V, but as it discharges, this voltage drops, perhaps down to 3.0V or lower. Sensitive electronics often require a stable, constant voltage to operate correctly. DC-DC converters can take this varying input and produce a steady output.
Efficiency is Key: You could use simpler methods like linear regulators or voltage dividers to drop voltage, but these are often incredibly inefficient, especially when there’s a large difference between the input and output voltage. They essentially burn off the excess voltage as heat. DC-DC converters, particularly switching converters (which we’ll focus on), can achieve efficiencies well over 90%, meaning less wasted energy, less heat generation, and longer battery life.
Stepping Up Voltage: Sometimes, you need a higher voltage than your source provides (like powering that phone backlight from a lower voltage battery). Simple resistive methods can’t do this, but certain DC-DC converters are specifically designed to “boost” the voltage.
So, What IS a DC-DC Converter?
At its core, a DC-DC converter is an electronic circuit that takes a DC voltage input and produces a different DC voltage output. This output can be lower (step-down), higher (step-up), or even inverted (positive to negative) relative to the input.
The most common and efficient types are Switching DC-DC Converters (often just called “switchers” or SMPS – Switched-Mode Power Supplies, although SMPS can also convert AC-DC). Unlike linear regulators that act like a variable resistor, switching converters work by rapidly turning a switch (usually a transistor like a MOSFET) on and off. This switching action, combined with energy storage elements like inductors and capacitors, allows them to transfer power efficiently from input to output, manipulating the voltage level in the process.
How Do They Work (The Basic Principle)?
Imagine you want to transfer water from a high-pressure hose (input voltage) to fill a bucket (output load) at a lower, controlled pressure (output voltage).
A linear regulator would be like putting a valve on the hose and just partially closing it, dissipating the excess pressure (energy) as friction (heat).
A switching converter is more like rapidly turning the hose fully on and fully off:
Switch ON (Energy Storage Phase): You turn the hose fully on for a short period. Water flows rapidly into an intermediate “storage tank” (this is analogous to an inductor storing energy in its magnetic field or a capacitor storing energy in its electric field).
Switch OFF (Energy Release Phase): You quickly turn the hose fully off. The water stored in the intermediate tank now flows out smoothly to fill the final bucket (the energy stored in the inductor/capacitor is released to the load).
Control: By carefully controlling how long the switch stays on versus off (this timing is called the duty cycle) and how frequently this switching happens (the switching frequency), you can precisely regulate the average voltage or current delivered to the output. A controller circuit constantly monitors the output voltage and adjusts the switching pattern to keep it stable, even if the input voltage or the load changes.
This rapid switching (often hundreds of kilohertz to several megahertz) is the key to their efficiency. The main power-handling switch is ideally either fully ON (low resistance, minimal voltage drop, low power loss) or fully OFF (no current flow, zero power loss). The energy storage elements (inductors and capacitors) are also relatively lossless compared to resistors.
The Main Flavors: Common Types of DC-DC Converters
While the underlying principle involves switching and energy storage, different arrangements of these components lead to different converter types, each suited for specific tasks. Here are the most fundamental non-isolated topologies:
Buck Converter (Step-Down):
Function: Produces an output voltage lower than the input voltage.
Analogy: The most direct application of the “fast switching tap” idea described above.
Use Case: Generating 3.3V or 1.8V for microcontrollers from a 5V or 12V input. Very common in point-of-load (PoL) regulation on motherboards.
Boost Converter (Step-Up):
Function: Produces an output voltage higher than the input voltage.
How (Simplified): Stores energy in an inductor when the switch is ON, then when the switch opens, the collapsing magnetic field forces this stored energy (along with the input voltage) out to the load at a higher voltage level.
Use Case: Powering LED strings (requiring maybe 20V) from a 12V battery, or generating a higher voltage rail in battery-powered devices.
Buck-Boost Converter (Step-Up/Step-Down):
Function: Can produce an output voltage that is either higher or lower than the input voltage. Often, the output voltage is also inverted (e.g., positive input gives negative output).
How (Simplified): Combines aspects of both Buck and Boost. Energy is stored in the inductor when the switch is ON and released to the load when the switch is OFF. The output voltage polarity is typically opposite to the input.
Use Case: Generating a negative voltage rail, or applications where the input voltage can swing above and below the desired output voltage (like a battery discharging). Variations like the SEPIC, Cuk, and Zeta converters can provide non-inverted buck-boost functionality.
Isolated vs. Non-Isolated Converters:
The types above (Buck, Boost, Buck-Boost) are typically non-isolated. This means there’s a direct electrical path (common ground) between the input and output.
For safety reasons (preventing high voltage input from reaching the user-accessible output) or to break ground loops and reduce noise, isolated DC-DC converters are used. These employ a transformer within the switching circuit to transfer energy magnetically while maintaining electrical isolation. Common isolated topologies include:
Flyback Converter: Simpler, often used for lower power (like phone chargers – AC-DC followed by DC-DC stage). Can be step-up or step-down.
Forward Converter: More complex than Flyback but generally more efficient and suitable for higher power levels. Typically step-down.
Push-Pull, Half-Bridge, Full-Bridge: Used for progressively higher power levels.
Key Parameters to Understand:
When looking at or selecting a DC-DC converter, several parameters are crucial:
Input Voltage Range (Vin): The range of DC voltages the converter can accept.
Output Voltage (Vout): The desired DC voltage the converter produces. Can be fixed or adjustable.
Output Current (Iout): The maximum current the converter can supply to the load.
Efficiency (%): Output Power / Input Power. Higher is better (less wasted heat). Typically varies with load and input voltage.
Switching Frequency (f_sw): How fast the internal switch operates. Higher frequencies generally allow for smaller inductors and capacitors (smaller overall size) but can sometimes lead to higher switching losses (lower efficiency).
Output Voltage Ripple: The small AC component remaining on the DC output voltage. Caused by the switching action and the charging/discharging of the output capacitor. Lower ripple is usually preferred for sensitive circuits.
Load Regulation: How much the output voltage changes when the load current changes.
Line Regulation: How much the output voltage changes when the input voltage changes.
Where Do We Find Them? Everywhere!
The applications of DC-DC converters are vast and growing:
Consumer Electronics: Smartphones, tablets, laptops, digital cameras, game consoles (generating core voltages, memory voltages, etc.).
Automotive: Powering infotainment systems, ECUs (Engine Control Units), LED lighting, charging ports from the car’s 12V or 48V system. Electric vehicles rely heavily on them for managing battery power.
Industrial: Power supplies for automation equipment, control systems, sensors.
Telecommunications: Powering base stations, routers, switches.
Medical Devices: Ensuring stable, reliable power for sensitive diagnostic and life-support equipment.
Renewable Energy: Solar power systems use MPPT (Maximum Power Point Tracking) converters (often Boost or Buck-Boost variants) to extract the maximum possible power from solar panels under varying conditions. Also used in wind turbines.
Aerospace and Defense: High-reliability power conversion in demanding environments.
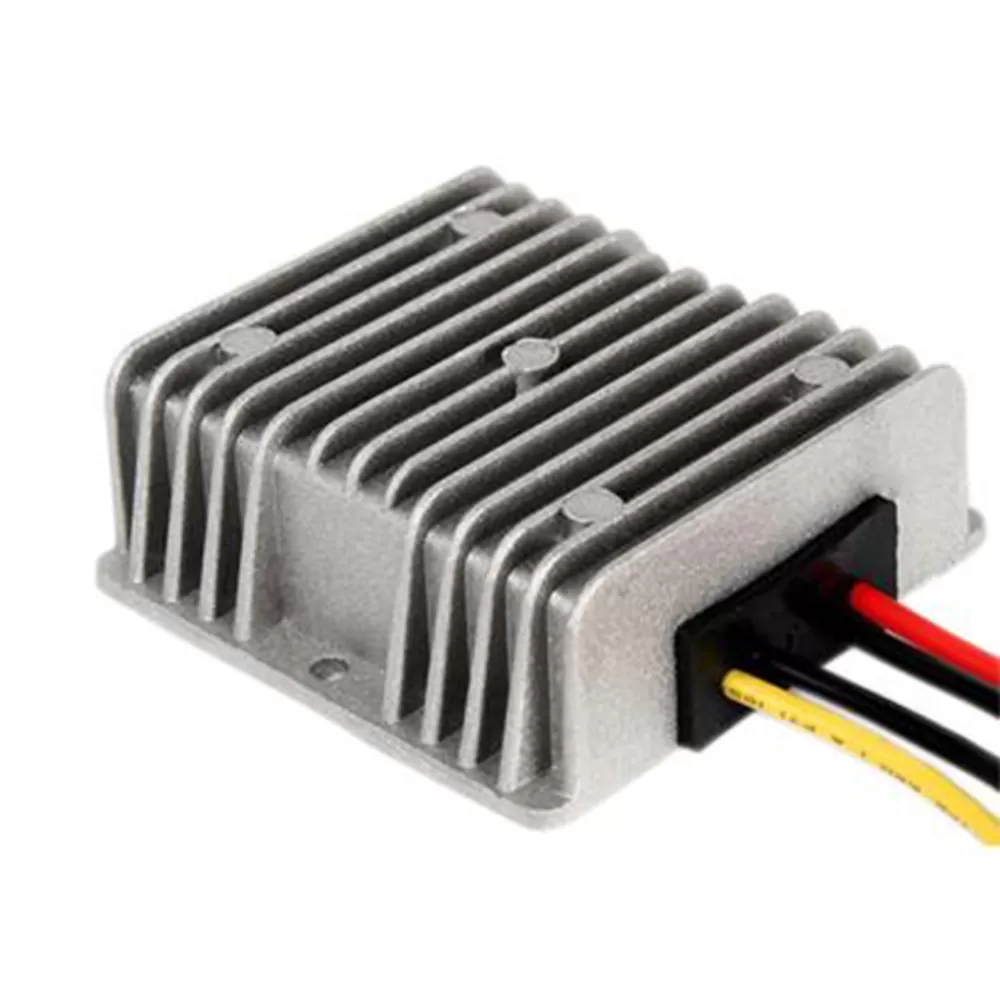
Conclusion: The Power Behind the Power
DC-DC converters are fundamental components that make our modern electronic world possible. By efficiently transforming DC voltage levels, they allow complex systems with diverse power requirements to operate from common sources like batteries or power buses. From stepping down voltage for delicate microprocessors to boosting it for bright LED displays, these switching circuits manage power with remarkable efficiency.
Understanding the basics of what they are, why they’re needed, and the general principle of how they work opens a window into the fascinating field of power electronics. The next time you charge your phone or marvel at the battery life of your laptop, remember the tiny, hardworking DC-DC converters inside, silently ensuring everything gets the power it needs, precisely when it needs it.
What are your thoughts? Do you have any specific questions about DC-DC converters, perhaps about a particular type or application? Let me know in the comments below!
I hope this draft provides a good starting point for your blog post. You can adjust the depth of technical detail, add diagrams (highly recommended for explaining topologies), or tailor the examples further based on the specific nuances you want to emphasize for your audience. Good luck!